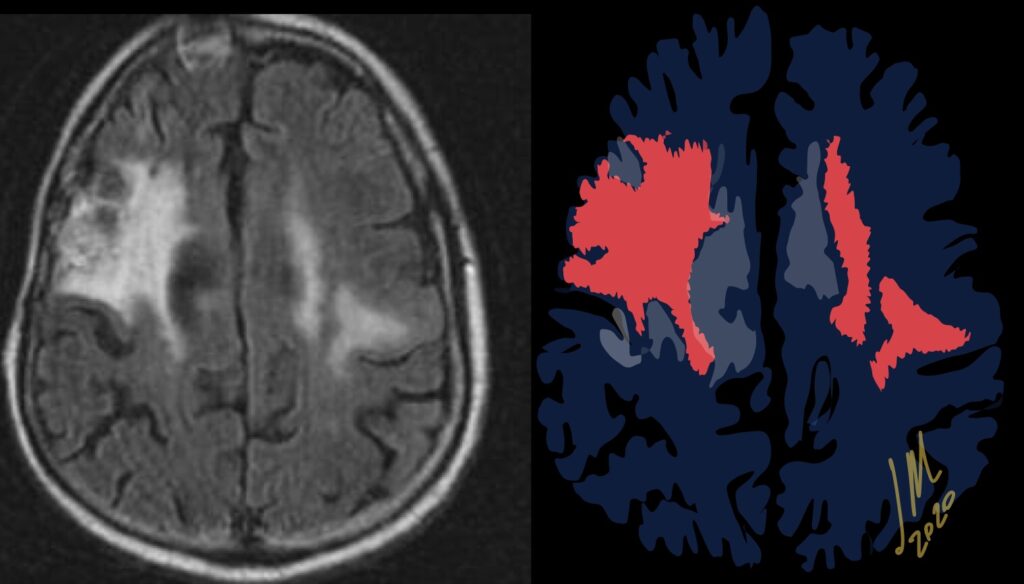
Cerebral radiation necrosis is a serious late delayed complication that manifests after a latency period of several months, although the range is broad and cases have been reported more than 10 years after irradiation. Histopathologic features of radiation necrosis include fibrinoid changes in the blood vessels, coagulative necrosis, demyelination, and gliosis. The disruption of the BBB (as visualized on gadolinium-enhanced images) may be mediated in part through vascular endothelial growth factor (VEGF) that is released in response to hypoxia. The reported incidence of radiation necrosis after RT for brain tumors ranges from 3% to 24%. Radiation necrosis is more likely to occur when high doses per fraction are administered, and combined use of chemotherapy with RT may play a role in development of treatment-related necrosis. Targeted therapy and immunotherapy may also increase the risk of treatment-related necrosis. Radiation necrosis typically develops at or adjacent to the original site of the tumor, the location that received the highest radiation dose. Radiation necrosis can also develop in part of the normal brain parenchyma that was included in the treatment field of a tumor outside the brain. In many cases, radiation necrosis is a self-limiting process that can be managed conservatively without intervention, although some patients need steroids for symptomatic relief.
Conventional MRI typically demonstrates an enhancing mass lesion with central necrosis and reactive edema. The enhancement pattern is often described as “spreading wavefront,” meaning that the margins of the enhancement are ill defined or “soap bubble–like” or “Swiss cheese–like,” meaning that the enhancing lesion includes central nonenhancing necrotic components of varying size. Multiple lesions are possible, and some lesions may develop distant from the original site of the tumor if the distant site was in the radiation field
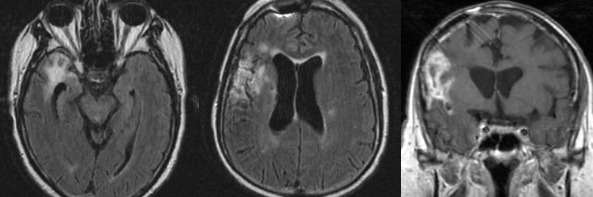
As radiation necrosis progresses with tumorlike growth, it can lead to marked shrinkage of the white matter and cortex and result in focal brain atrophy. The periventricular white matter is among the areas most susceptible to radiation necrosis. This may be explained by this neuroanatomic region having relatively poor blood supply from long medullary arteries that lack collateral vessels, making it vulnerable to ischemic effects produced by postirradiation vasculopathy. Imaging findings of radiation necrosis are not always irreversible and progressive but can be static, decrease, or even resolve at follow-up . White matter lesions were the earliest and most common manifestation after RT, followed by enhancing lesions, which often became necrotic with increasing size. Both white matter lesions and enhancing lesions were more likely than cysts to regress, and both could show complete resolution. Cysts were the least frequent pattern of radiation-induced injury and developed from enhancing lesions that exhibited necrosis
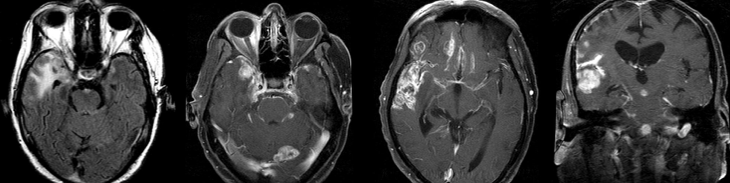
Radiologic features of radiation necrosis at conventional imaging overlap with those of recurrent tumors, including high-grade primary brain tumors and brain metastases; therefore, image interpretation can be challenging. Biopsy of the suspicious lesion may be required for a definitive diagnosis, particularly in patients who are symptomatic and have worsening imaging findings over time. However, it is known that even at histopathologic analysis, residual or recurrent tumor mixed with radiation necrosis is a common finding.
Although not always reliable, some clinical or imaging features have been suggested in previous reports and may aid in diagnosis of radiation necrosis and tumor recurrence. First is the time elapsed since RT. Radiation necrosis usually manifests after a latency period of many months (12 months average) a new or worsening abnormality starting 3 years after RT is unlikely to be due to pure radiation necrosis. Corpus callosum involvement in conjunction with multiple enhanced lesions—with or without crossing of the midline and subependymal spread—were statistically significant, favoring predominant glioma recurrence
MR spectroscopy attempts to identify tumor recurrence by providing information on metabolic changes. Recurrent brain tumors exhibit high ratios of choline/creatine (Cho/Cr) and choline/N-acetylaspartate (Cho/NAA) whereas radiation necrosis exhibits increased lactate and lipid peaks .
Dynamic susceptibility contrast (DSC) MRI is the most widely used perfusion technique for brain tumors. DSC perfusion MRI relies on the T2- and T2*-shortening effects of gadolinium-based contrast agents and involves rapid imaging to capture the signal intensity changes due to the first passage of an intravenously administered contrast agent bolus.
The main parameter derived from DSC MRI in the context of brain tumors is relative cerebral blood volume (rCBV). rCBV is an indirect index of cerebral blood volume, generally calculated relative to region of interest (ROI) values in the contralateral normal white matter. Radiation necrosis typically causes hypoperfusion with reduced rCBV, whereas high-grade tumor recurrence results in high rCBV.
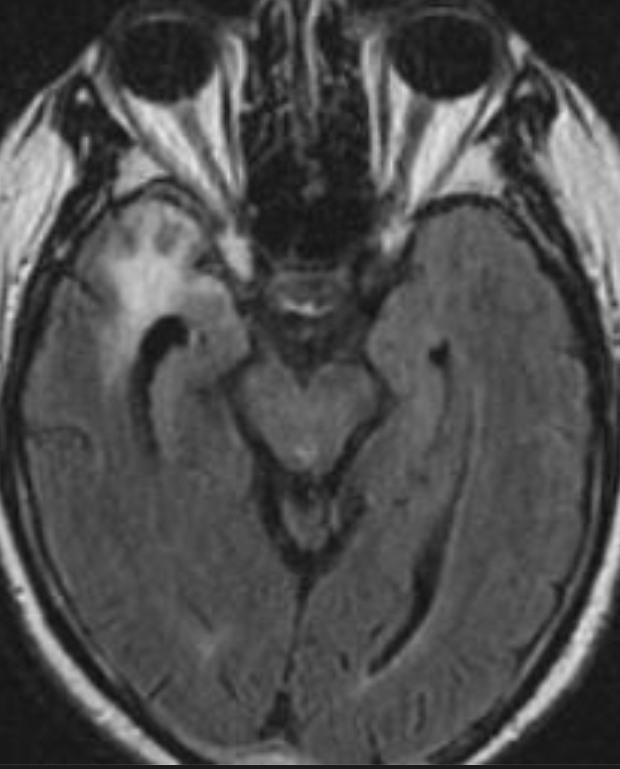
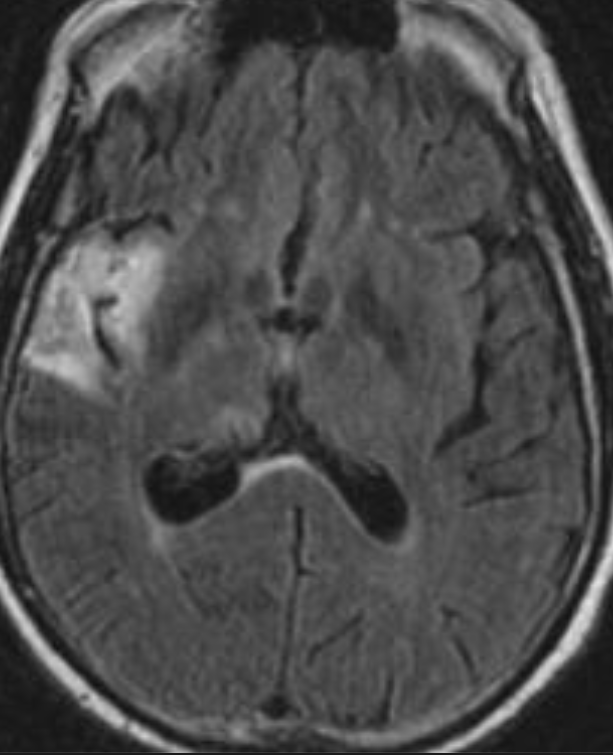
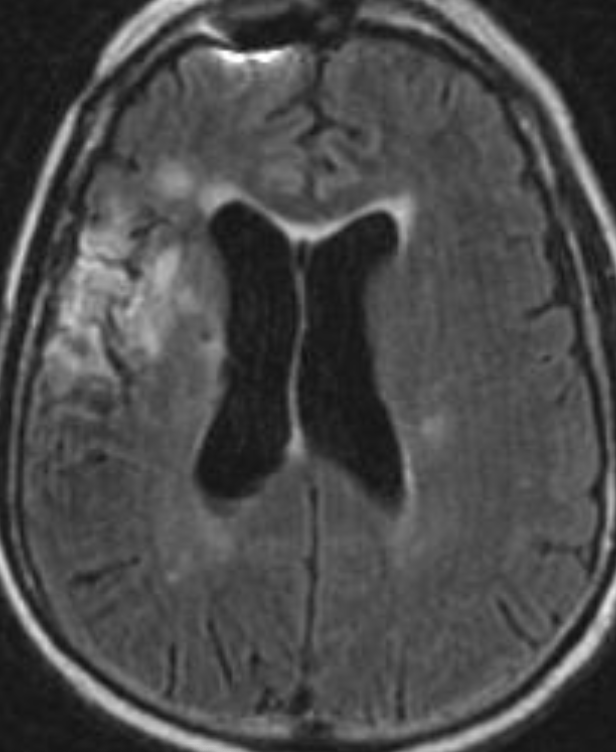
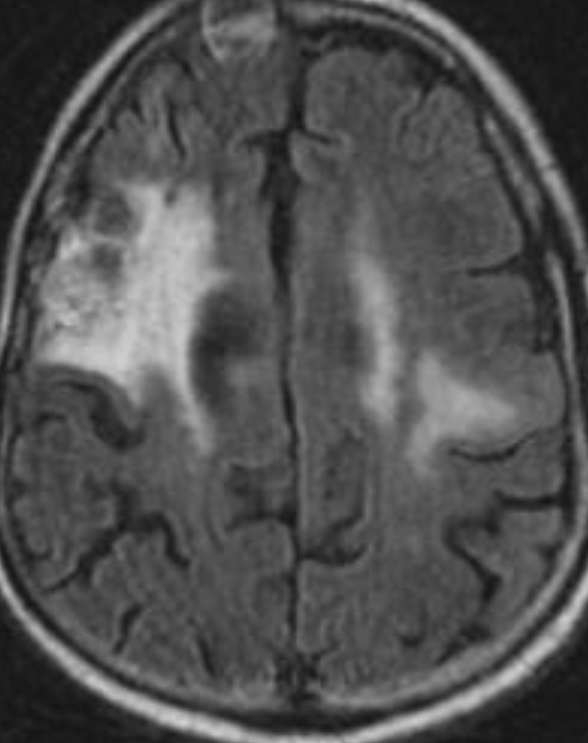
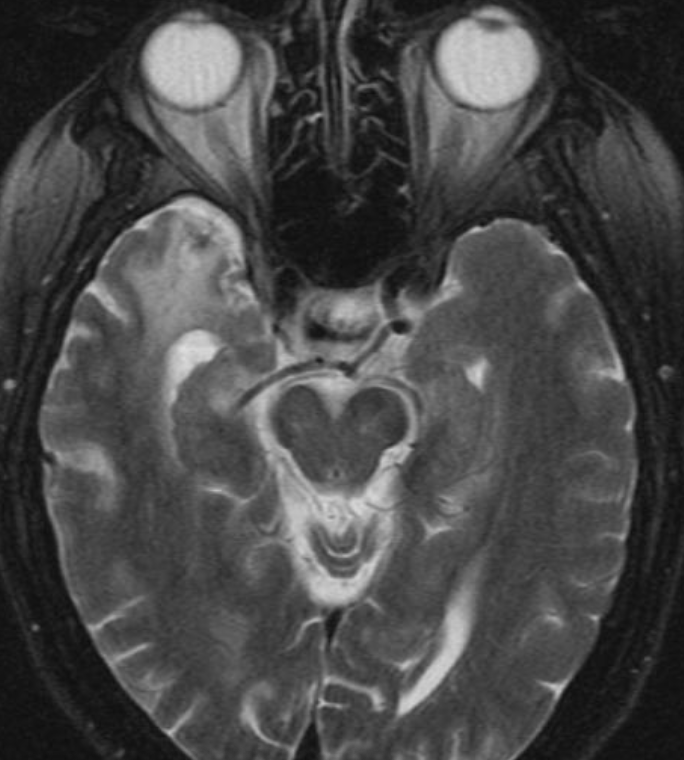
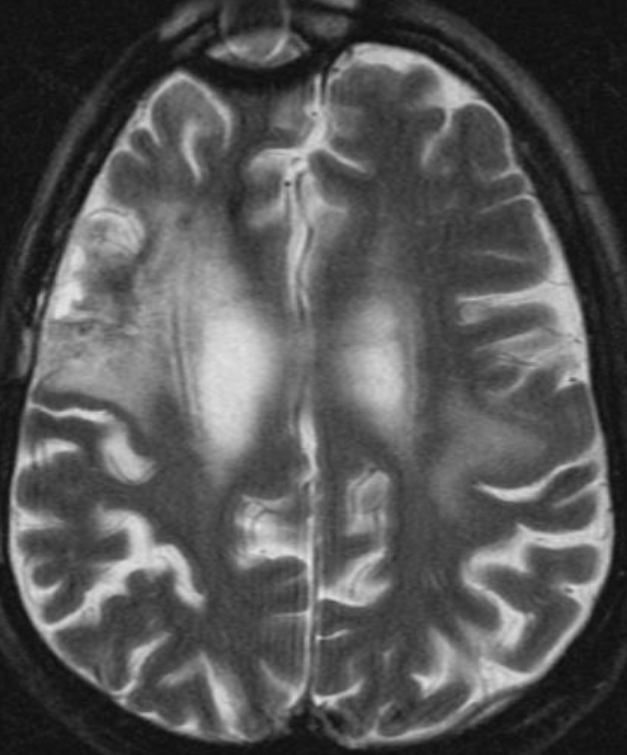
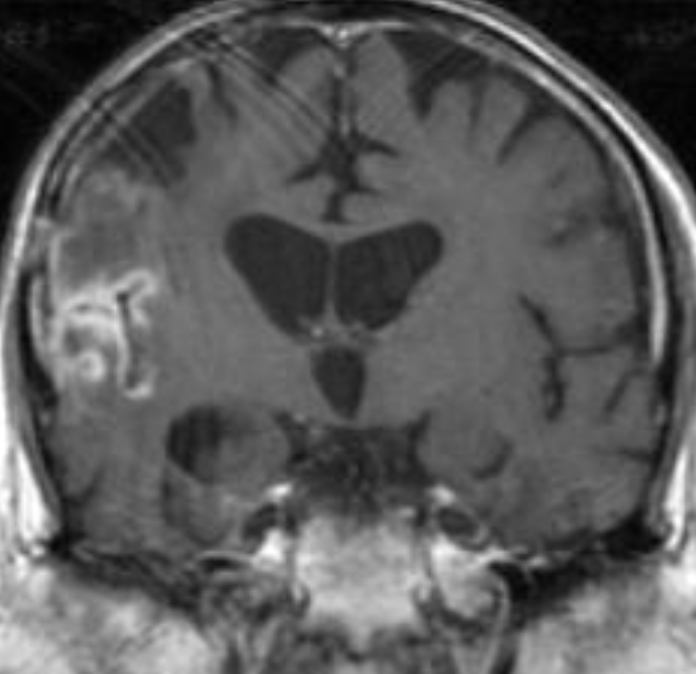
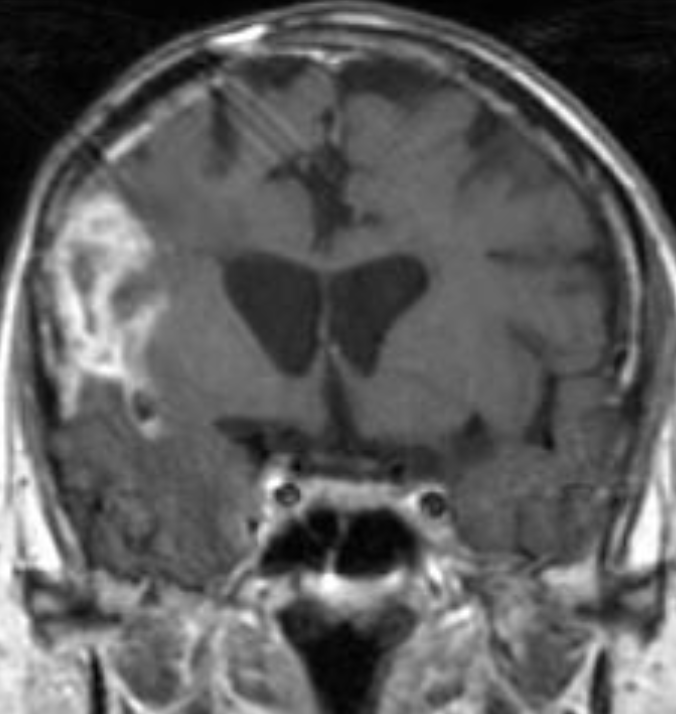
The only method of distinguishing pseudoprogression and true tumor progression is to perform follow-up examinations of the patient because conventional MRI does not allow differentiation of the two conditions. Imaging may be regularly performed at 2–3-month intervals throughout the follow-up period although the frequency of imaging can be variable across institutions. In clinical practice, the following features can be helpful: (a) presence of symptoms and (b) methylation status of the MGMT gene promoter.
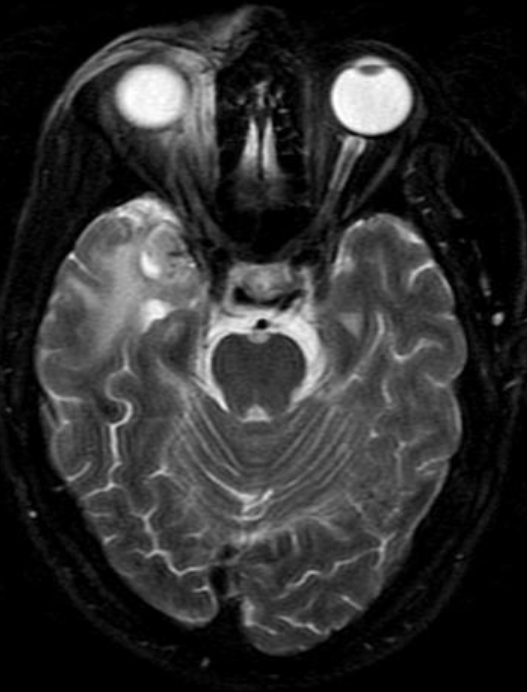
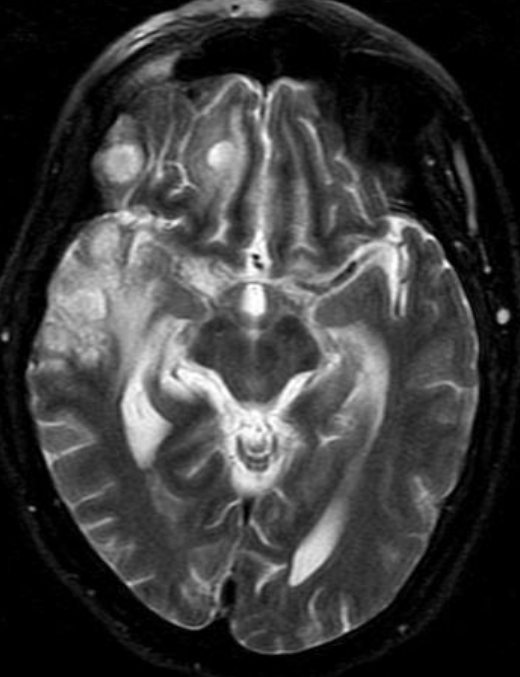
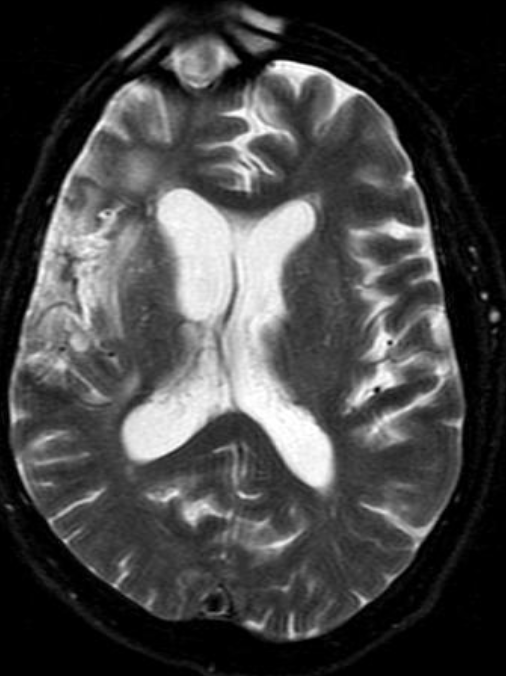
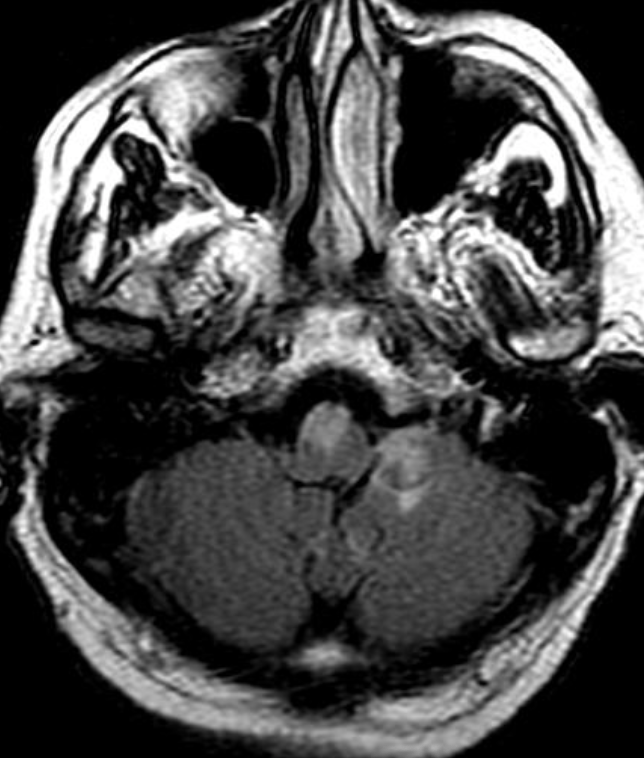
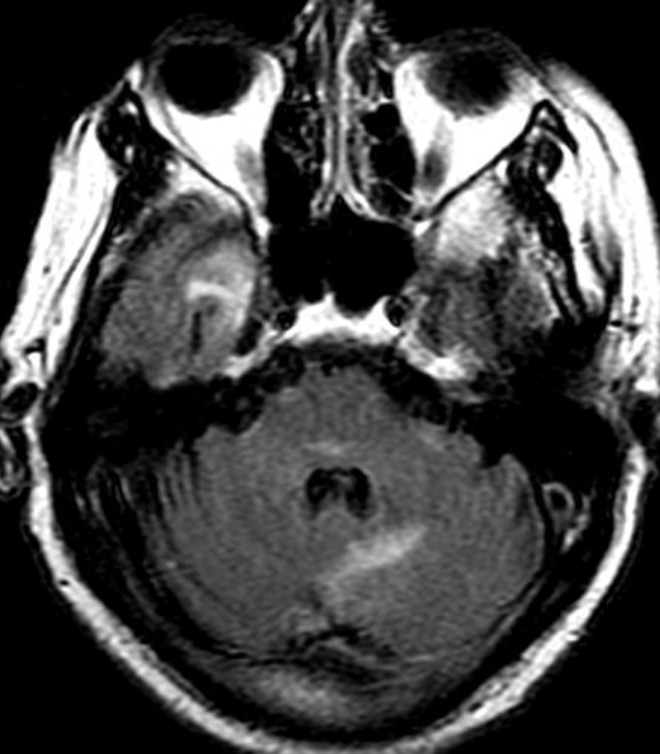
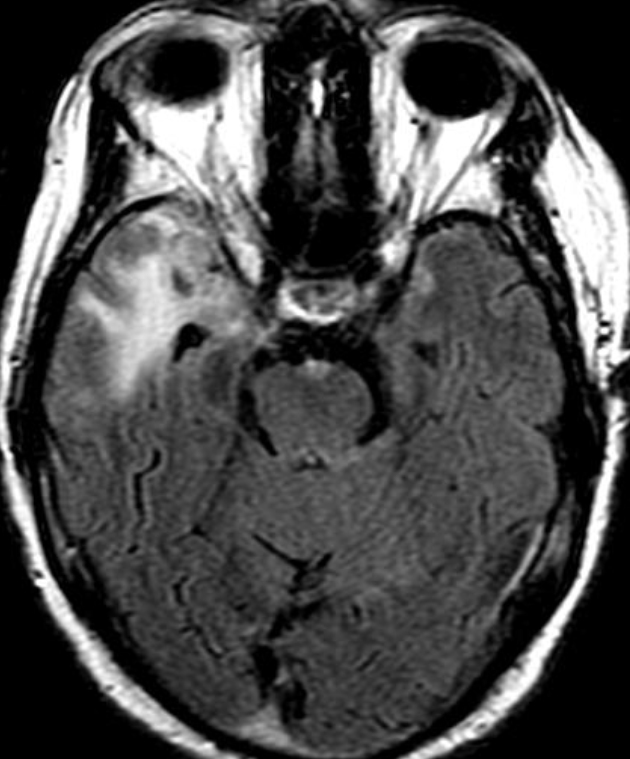
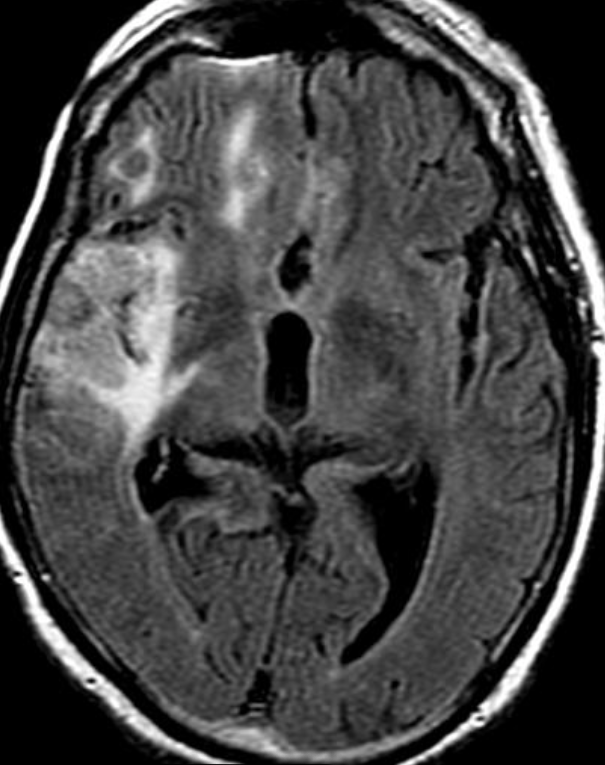
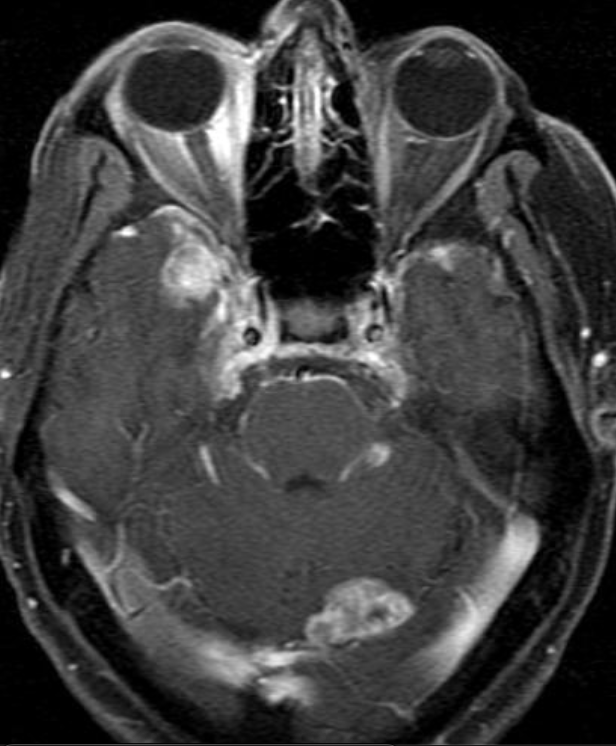
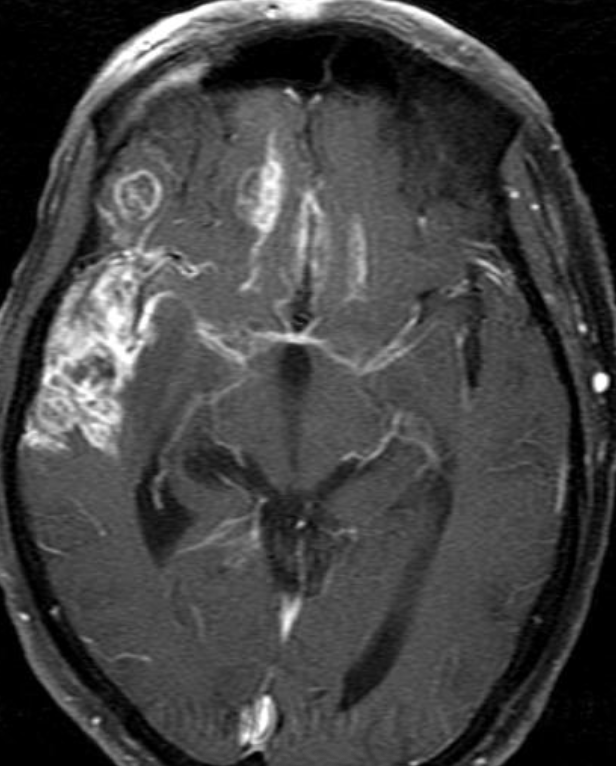
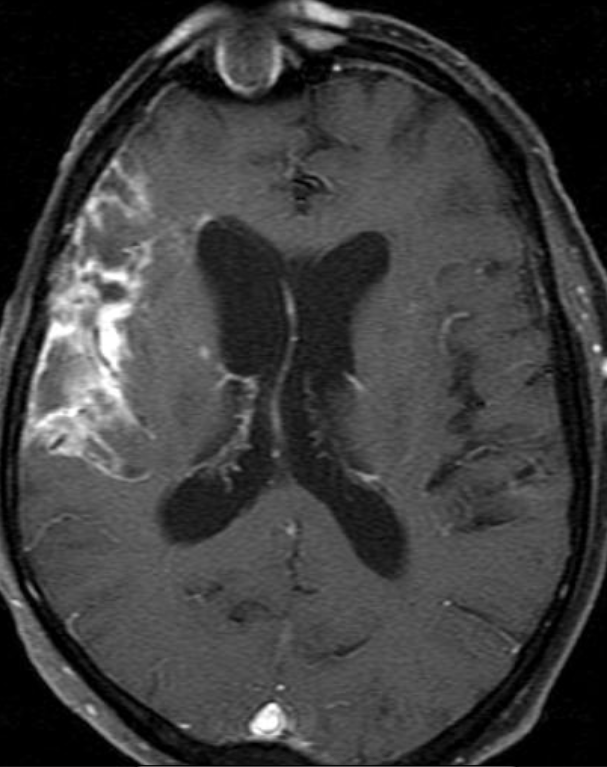
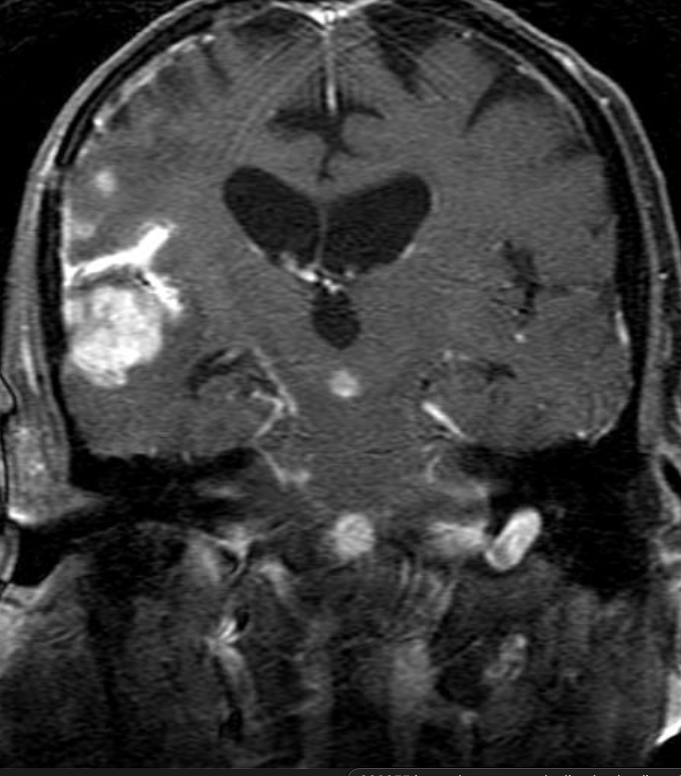
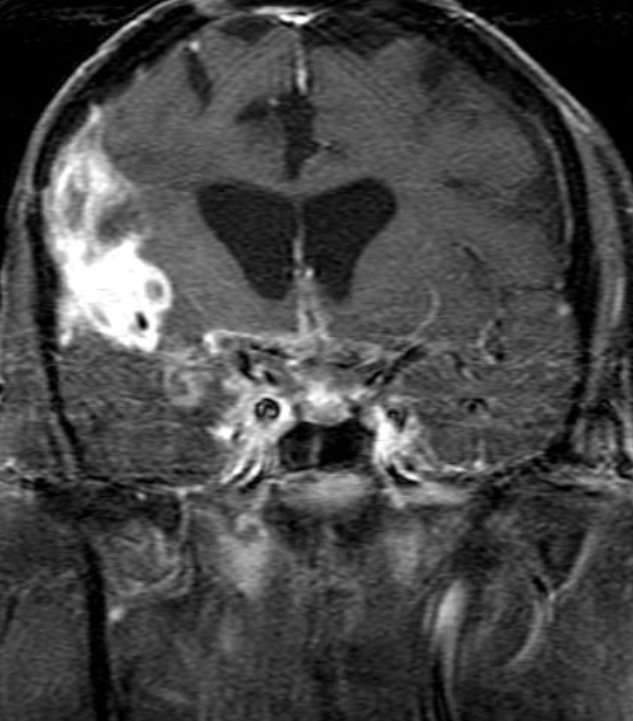
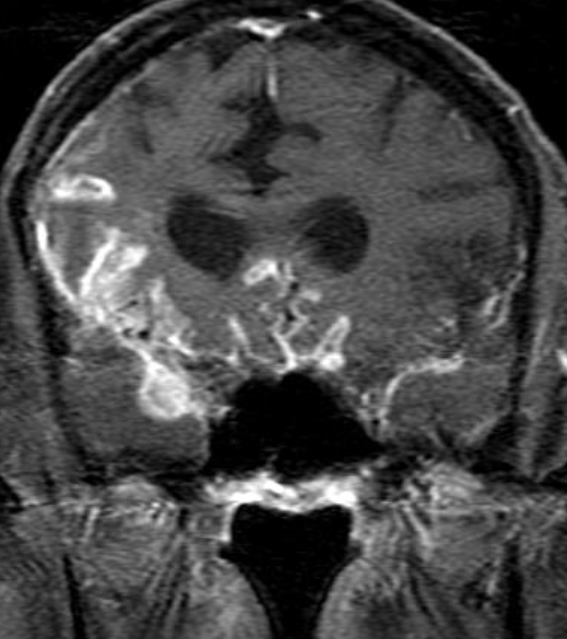
In the central nervous system, the effects of radiation can be roughly divided into effects on vascular endothelial cells and direct effects on neuroglial cells, in particular the oligodendroglial cells.
Vascular endothelial damage causes altered permeability, leading to vasogenic edema and disruption of the blood-brain barrier (BBB) or blood–spinal cord barrier. Preclinical studies suggest that endothelial damage may occur within the first 24 hours after irradiation. Endothelial damage can lead to other late vascular effects, such as telangiectasia, thrombosis, occlusion of small vessels, fibrinoid deposits, and hyaline thickening of vessel walls. As a result, ischemic stroke or hemorrhage may occur months to years after RT.
Oligodendrocytes are the most radiosensitive type of glial cell, with cell death occurring rather early after relatively low doses of irradiation. Radiation not only reduces the number of mature oligodendrocytes but also induces loss of their precursors, the oligodendrocyte type 2 astrocyte. This results in failure to replace normally turned over oligodendrocytes, with the eventual consequence being demyelination. In addition, there are changes in cellular composition such as increased numbers of reactive astrocytes (gliosis) and microglia. These reactive cells have been reported to produce reactive oxygen species, proinflammatory cytokines, and growth factors (eg, vascular endothelial growth factor [VEGF]), leading to a cycle of further cellular toxic effects and tissue damage .
Radiation-induced injury can be divided into three phases: acute, early delayed and late delayed. Acute and early delayed injuries are usually transient and reversible, whereas late delayed injuries are generally irreversible. Keys to recognizing radiation-induced changes at follow-up imaging are knowledge of (a) the amount of time elapsed since RT, (b) the location of the target lesion, and (c) the amount of normal structures included.
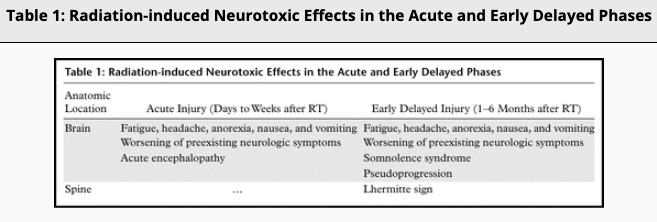
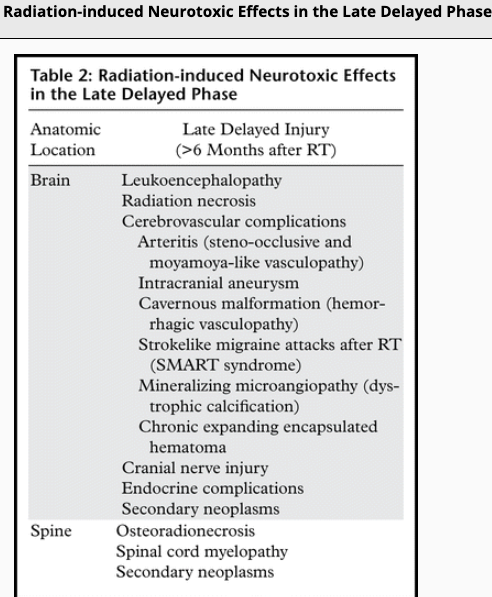
Reference: Published Online:Nov 20 2020 https://doi.org/10.1148/rg.2021200064